Understanding the Standard Model
Today I want to talk high level about the Standard Model and
try to extract its insights. The Standard Model is basically quantum field theory and there are excellent books
available on the topic. Personally
I learned QED long ago from
Mandl and Shaw – an excellently balanced and clear
introductory textbook (I never read the second edition). If you want to really understand what is going on and not
miss the forest because of the trees, an outstanding book is Zee’s "
Quantum Field Theory in a nutshell” (maybe in a watermelon-the book has 518 pages). However, I do not recommend it as a first book but read it only after reading the first 10 chapters of Mandl and Shaw. For the serious
practitioner earning a living computing Feynman diagrams,
Peskin and Schroeder
is the gold reference.
Mathematically, the basic idea is that of fiber bundles: just think of them
as a common rug. However, Zee has a much better pictorial representation for physicists: consider the space-time like a giant mattress.
Jump on it and you create a particle (excitation) at that coordinate. Of course you have bosons and fermions. Let's discuss the simplest case: the electron and electromagnetism. Let's forget about spin and spinors for the moment. The probability to detect the electron is given by the quantum wavefunction which is attaches a complex value at each space-time point. All expectation values are insensitive to an overall complex number phase, and by
Noether theorem, invariance under this symmetry implies a law of conservation: the charge is conserved. Now let's add relativity which demands that signals cannot go faster than the speed of light. However this is at odds with the uncertainty principle and the way out is to go to
second quantization which demands pair production of particles and anti-particles. If the
global symmetry is changed into a
local symmetry, we get to the idea of fiber bundles and local phase changes demand an adjustment in computing derivations. In other words we have what mathematicians call a
connection and physicists call a
potential. The potential turns out to be a vector potential \(A_\mu \) and this is the electromagnetic potential. Upon quantization, the picture now becomes that of
Feynman diagrams: the vector potential which comes from a local phase mismatch between neighboring points is now a virtual photon in Feynman diagram.
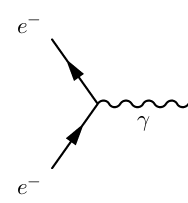
So this is the basic idea. One more thing I learned in graduate school is that such diagrams were known before Feynman, but they were not computed relativistically. Feynman major contribution was to compute them relativistically which introduced about an order of magnitude overall simplification in their computation. As an apocryphal story, Feynman once attended a physics conference where someone presented the result of six month of computation using non-relativistic diagrams which Feynman managed to double check in thirty minutes using his method and found a mistake.
Now electromagnetism is known to be invariant under gauge transformations, and the way you couple the electron to the photon (known as
minimal coupling) preserves this gauge invariance.
So far so good, electromagnetism is an interaction based on exchanging a particle (a virtual photon) and since the photon is massless, the range of the interaction is infinite. How about the other interactions? It turns out all other interactions are basically the same thing and
electromagnetism generalizes into Yang-Mills gauge interactions. Here is how is done: In quantum mechanics there is a 1-to-1 correspondence between observables and generators. Observables are hermitian operators which obey a Jordan algebras, while generators are anti-Hermitean operators which obey a Lie algebra. From generators of Lie algebras one constructs by exponentiation a Lie group and in this case we are talking about \( SU(n) \). Q: how many generators are for \( SU(n) \)? A: \(n^2 - 1\). For electromagnetism the gauge group is \( U(1) \) which has elements of the form \( e^{i \phi} \), the weak force has three generators (the Pauli matrices), and the strong force has eight generators.
The generators correspond in second quantization to emission and absorption of one quanta of interaction (photon, W+, W-, Z, 8 gluons) and they are 4-vectors just like \( A_\mu \). The key difference between electromagnetism and Yang-Mills is that the generators do not commute. Why? Expressed them as matrices: \( A_\mu = (A_0, A_x, A_y, A_z ) \) where each \(A \) is a \( n \times n\) matrix. Physically this means that they carry "charge". In electromagnetism there is only one electric charge, in weak interaction there are two charges (which mixes electrons with neutrinos and up quarks with down quarks), and in the strong interaction there are three charges (red, green, and blue). Alternatively, an electron or a neutrino is the same physical particle which becomes an electron or a nutrino upon measurement just like an electron has a spin which becomes up or down when passed through a Stern-Gerlach device. Because the field lines carry charge, unlike in electromagnetism, for two charges in the \( SU(3) \) and higher hypothetical \( SU(n>3)\) cases the field lines are parallel because it is energetically more advantageous. What this meas is that quarks cannot be free because separating them adds energy to the point where two more quark-antiquark particles are formed in the middle. For the strong interaction the only possible states which are allowed in nature are the singlet state of zero color charge, all other states requiring an infinite amount of energy to be created. For \(SU(3)\) there are only two possible singlet color states corresponding to 3 or 2 quarks (proton, neutron for 3 quarks, mesons for 2 quarks).
The gauge group of the Standard Model is \(U(1)\times SU(2) \times SU(3) \) which means that the following particles are possible:
\(up_{r} ~~~ down_{r}\)
\(up_{g} ~~~ down_{g}\)
\(up_{b} ~~~ down_{b}\)
\(e ~~~ \nu\)
The strong force mixes the top three rows, while the weak force mixes the two columns. The particles here form what is called a "
family". There are two more families identical from the point of view of gauge symmetries, but different in mass (heavier). The origin of the families is unknown and a possible explanation comes from string theory.
Now all the particles (photon, Ws, Z. gluons, electrons, neutrinos, quarks) are massless or nearly massless (compared with the energy level at the unification scale which is the natural energy).
Adding mass to photons, Ws, Z, gluons in the Lagrangian spoils gauge invariance, but this can be restored if it is part of another field called the Higgs field. How does this work? A zero mass particle, just like the photon has two degrees of freedom corresponding to two perpendicular modes of oscillation (two polarizations). Making a massless particle into a massive one adds a longitudinal degree of freedom which must come from some other field. If you recall the "
Mexican Hat potential", a Higgs field has two modes of oscillation: radially (corresponding to the Higgs particle) and circular corresponding to a massless Goldstone boson. The
Goldstone boson combines with a massless particle like W and gives rise to a massive W. This is why W and Z particles are massive and because of it particle decay is relatively slow. How do particle decay? Take a heavy quark-antiquark combination, they form a W particle in a mechanism similar with vacuum polarization is QED and then W decays into a lighter combination of electron and antineutrino.
The Higgs mechanism works only for W+, W-, and Z, not for photons or gluons. There are two more twists in the Higgs process. First, this mechanism breaks the symmetry but the singlet boson mixes with the electromagnetism boson and results in the massive Z and the massless photon. The photon is not really the photon before symmetry breaking! Second, how do the fermions acquire mass?
Fermions interact with other fermions through the minimal coupling from above. However, they also couple with the Higgs field by "
Yukawa coupling" and this is how they get mass. Fermion masses has to do with the fact that in nature the mirror symmetry is broken. Left handed particle behave differently than right handed particle in weak interaction. If you look at Dirac's equation and write it in terms of left handed and right handed components, the fermion propagation mixes them up. Without Yukawa coupling for fermions the left-handed physics would have been completely equivalent with right handed physics. What happens is that
Dirac's equation is valid only in the approximation that the Higgs field does not excite radial oscillations and the mass of the fermions depend on the radial value of the Mexican Hat potential at the minima and the coupling coefficient.
Now if the Higgs Mexican hat potential would have had a different minima, or if the Yukawa coupling would have been different, then our universe would have looked completely different. Take the up and down quark masses for example. If those masses would have been the other way around, then the neutron would have been stable and the proton would have decayed into it preventing the formation of atoms. Chemistry and life would have been impossible.
Our universe is the way it is because we are trapped in a local energy minima. What generates this minima? Can an ant walking on the surface of an apple understand the concept of the apple and how it got there? I think one of the triumphs of string theory is actually predicting the
landscape of local minima despite the criticism of the lack of predictive power. This is the
Copernican principle in action. We are in no way special. The Earth is one of the planets of the solar system, the Sun is one of the 100 billion stars in out galaxy, the Milky Way is one of the 100 billion known galaxies, and probably our universe is one of the \( 10^{100}\) possible local energy minima each with very different particles then the ones in our universe. Quantum mechanics "multiverse" (from
MWI) is almost sure gobbledygook, but eternal inflation, bubble universes, and the multiverse landscape are almost surely real.